HPTLC is a highly versatile, reliable and cost-efficient tool for the rapid in-parallel analysis of multiple samples. Detection of adulteration, identification and purity tests, monitoring stability, and quantification of marker compounds, are fully exploiting the strengths of the technique in the analysis of matrix-rich samples. HPTLC can easily deal with complex and diverse food matrices and is typically used for quality control purposes, to test for additives and to screen for food contaminants. The complexity and diversity of samples in which analytes such as carbohydrates, lipids, proteins, vitamins, and minerals have to be identified and quantified, are a major challenge in food analysis. HPTLC offers high matrix tolerance, allowing rapid authentication and accurate quantification of target analytes in food samples.
Quality control of honey
Honey is a natural mixture of glucose and fructose with many other minor substances. Due to the high price of honey, particularly of mono-floral types, adulteration with other sugars and syrups is often observed in the market. The type and ratio of mono and oligosaccharides in a sample can also provide information about source, handling, and storage of the honey. The quantification of sugars is challenging due to their high polarity, low volatility, and lack of a chromophore. Their common occurrence in complex matrices, may require separation from proteins, lipids, and/or minerals as well as from other matrix constituents prior to analysis. HPTLC can effectively separate and sensitively quantify mono- and oligosaccharides in honey [1].
Conclusion
HPTLC is the method of choice for the analysis of honey, allowing the identification of the floral source, quantification of sugars as well as detection of sugar adulterants in honey.
Screening for aflatoxins in tomato extracts
Aflatoxins are natural mycotoxins produced by Aspergillus fungi. The fungal contamination of crops, nuts, dried and fresh fruits/vegetables is quite common, whereas high temperatures and humidity favor the occurrence of molds and thus the production of aflatoxins, which are known to be highly genotoxic and carcinogenic to humans and therefore must be controlled and prevented from use in food products. HPTLC rapidly identifies and reproducibly quantifies aflatoxins in food samples.
Conclusion
HPTLC is suitable for the quantification of aflatoxins B1, G1, B2, and G2 in tomato extract according to the Test for Aflatoxins of USP chapter <561> Articles of Botanical Origin, which limits aflatoxin B1 to 5 ppb and the sum of B1, G1, B2, and G2 to 20 ppb.
Analysis of illegal dyes in spices
Synthetically manufactured azo dyes are often illegally used for the artificial enhancement of the natural color of spices. Classified as carcinogens, their use as food additives is prohibited in the EU and the United States. Yet they are still used to amplify the color intensity of spices, particularly in countries in which the spices originate. Products offered as non-branded spices as they are available in public and food markets bear a higher risk of adulteration with illegal dyes. HPTLC is suitable for the rapid, sensitive, and reproducible analysis of spices contaminated with illegal dyes [3].
Conclusion
HPTLC is suitable for the reliable identification and accurate quantification of azo dyes in chili, paprika, curry powder and spice mixtures. Moreover, HPTLC is commonly used for dyestuff analysis in forensic, industrial and other applications.
Analysis of milk
Milk is a healthy and nutritious dairy product, consumed by a majority of the world's population. Among dairy products, human milk is particularly known for its presence of oligosaccharides (HMOs – Human Milk Oligosaccharides) because they are minimally digested in the gastrointestinal tract and reach the colon intact, where they shape the microbiota. Oligosaccharides are important components containing a group of structurally complex, unconjugated glycans. HPTLC is well suited for detection HMOs component, such as for in-process control during fermentation, or for monitoring of purification steps, and QC of finished products like HMOs. All production cycles can be followed by using the same methodology [4].
Milk products such as milk powders are sometimes affected adulteration for economic reasons. The practice of adulterating milk invariably reduces its quality and can introduce harmful substances into the dairy supply chain, thus endangering the health of consumers. In 2008, melamine, found in infant milk products, caused kidney damage and several deaths among children. Melamine (1,3,5-triazine-2,4,6-triamine) may have been illegally added to mask low protein content in fraudulently diluted or low quality milk. Since then, there is a great need for rapid and reliable methods for quality control of milks [5].
Conclusion
In milk, a very complex mixture, HPTLC is suitable for the screening and quantification constituents such as oligosaccharides. Melamine, a dangerous adulterant can be detected with a limit of 20 mg/L.
Analysis of polyphenols in coffee beans [6]
Coffee beans are a rich source of bioactive phytochemicals such as chlorogenic acids (CGA), including caffeoylquinic acids (CQA), feruloylquinic acids (FQA) and dicaffeoylquinic acids. Because during the roasting process, the chlorogenic acids content changes dramatically, a HPTLC method is used to follow the evolution of chlorogenic acids throughout the process also with the aim of controlling the roasting degree. The HPTLC chromatograms indicate similarities and differences in the composition of the identified compounds during the roasting process. Here, 3-CQA, 4-CQA and 5-CQA show light-blue fluorescence zones, whereby 4-FQA and 5-FQA show deep-blue fluorescence zones and the three dicaffeoylquinic acids like 3,4-di-CQA, 3,5- di-CQA and 4,5-di-CQA show light-green fluorescence zones.
Conclusion
The present study underlines the usefulness of HPTLC as a reliable tool to assess quality and quantity parameters of the coffee roasting process.
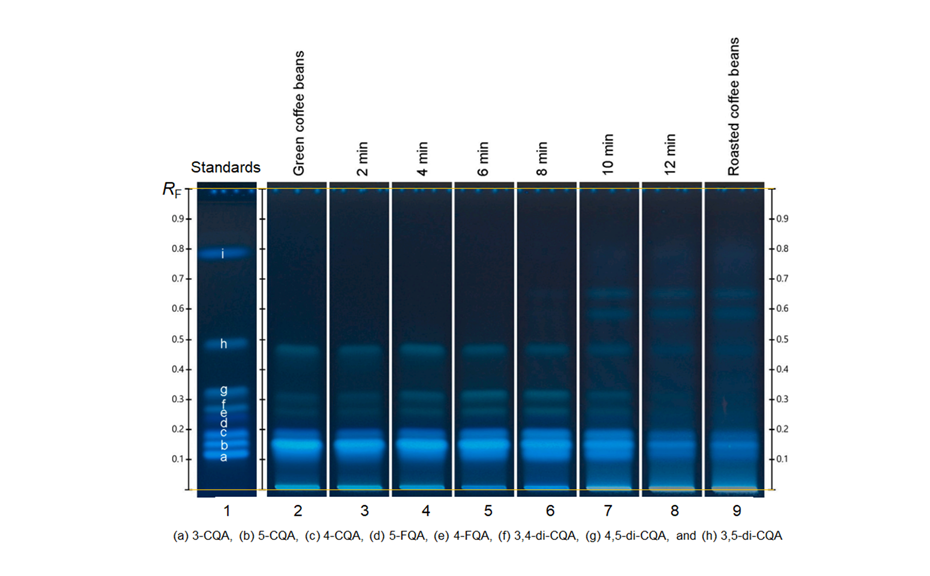
Figure 11: Comparative HPTLC fingerprints of the whole roasting process. Left track is the coffee mixtures for CGA with the separated compounds (a) 3-CQA, (b) 5-CQA, (c) 4-CQA, (d) 5-FQA, (e) 4-FQA, (f) 3,4-di-CQA, (g) 4,5-di-CQA, and (h) 3,5-di-CQA illuminated with UV 366 nm, derivatization with NPA reagent
Literature
[1] M.K Islam, T. Sostaric, L.Y. Lim, K. Hammer, C. Locher (2020) Sugar Profiling of Honeys for Authentication and Detection of Adulterants Using High-Performance Thin Layer Chromatography, Molecules 2020, 25, 5289. https://doi.org/10.3390/molecules25225289
[2] https://www.hptlc-association.org/methods/methods_for_identification_of_herbals.cfm
[3] H. Kandler, M. Bleisch, V. Widmer & E. Reich (2009) A Validated HPTLC Method for the Determination of Illegal Dyes in Spices and Spice Mixtures, Journal of Liquid Chromatography & Related Technologies, 32:9, 1273-1288, https://doi.org/10.1080/10826070902858293
[4] https://www.camag.com/sites/default/files/application_notes/A-139.1.pdf
[5] https://www.camag.com/sites/default/files/application_notes/A-88.1.pdf
[6] V. Pedan, E. Stamm, T. Do, M. Holinger, E. Reich, HPTLC fingerprint profile analysis of coffee polyphenols during different roast trials, Journal of Food Composition and Analysis, Volume 94, 2020, 103610, ISSN 0889-1575, https://doi.org/10.1016/j.jfca.2020.103610