Mercedes G. López and Luis F. Salomé work on agave fructooligosaccharides (aFOS, fructans) at the Research and Advanced Studies Center (CINVESTAV)-IPN in Mexico. Patricia A. Santiago and Ruth E. Márquez also conduct fructan research at the Interdisciplinary Research Center for the Integral Regional Development (CIIDIR)-IPN in Mexico. The group focuses on fructan chemical characterization and their biological effects as prebiotics on the human health.
Introduction
Fructans are a polydisperse mixture of fructose polymers, and contain only one or no glucose in their structures. They are commonly found in agaves and possess several industrial applications. These molecules have been mainly used as prebiotics and supplements to produce functional foods. Moreover, they are directly correlated with the yield and quality of the alcoholic drink tequila. The most powerful analytical technique for the characterization of fructans is high performance anion exchange chromatography (HPAEC). However, this technique is time consuming taking up to 80 min for a single sample. In this context, the HPTLC technique allows the parallel analysis of up to 17 samples and there are several options of derivatizing reagents for carbohydrate visualization. The solvent consumption is only 70 mL per sample batch, which is climate friendly.
Thus, this study aimed at exploring the potential of the aFOS fraction as a good descriptor of the fructan differentiation in agave species through age, and at the feasibility of HPTLC as a robust fingerprinting platform through multivariate data analysis (MVDA). In this study, HPAEC was used a standard technique for comparison with HPTLC.
The proposed method is rapid, accurate and precise. It is suited as a high-throughput method with a significant reduction in working time, supplies and solvents. Finally, it produces robust data which can be used for multivariate modelling.
Standard solutions
2.0 mg of glucose, fructose, sucrose, 1-kestose, 1-nystose, and 1-F fructofuranosylnystose (DP5) are dissolved in ethanol – water 7:3 (V/V).
Sample preparation
Agave fibers (Agave potatorum and Agave angustifolia) are extracted once in aqueous ethanol (80 %) for 1 h at 60 ºC, then reextracted twice with pure water. The extracts are defatted with chloroform and the aqueous phase is reduced and spray-dried. Samples are prepared at 7.0 mg/mL. They are firstly dissolved in 0.3 mL of water and then filled to 1.0 mL with absolute ethanol (room temperature).
Chromatogram layer
HPTLC plates silica gel 60 F254 (Merck), 20 x 10 cm are used.
Sample application
Samples and standard solutions are applied as bands with the Automatic TLC Sampler (ATS 4), 17 tracks, band length 6.0 mm, distance from left edge 20.0 mm, distance from lower edge 10.0 mm and 10.0 mm between bands. 5.0 µL for sample solutions and 1.0 µL for standard solutions are applied.
Chromatography
Plates are developed in the ADC 2 with chamber saturation (with filter paper) 20 min and after activation at 47% relative humidity for 10 min using a saturated solution of potassium thiocyanate. The first development is performed with isopropanol – butanol – water - acetic acid 14:10:4:2 (V/V) to the migration distance of 75 mm (from the lower edge), followed by drying for 5 min. The second development is performed with isopropanol – butanol – water - formic acid - acetic acid 14:10:4:1:1 (V/V) to the migration distance of 85 mm (from the lower edge), followed by drying for 5 min.
Post-chromatographic derivatization
The plates are immersed into a solution of diphenylamine-aniline-phosphoric acid (referred to as aniline) using the Chromatogram Immersion Device, immersion speed 2 cm/s and immersion time 2 s, dried for 30 s with cold air and heated at 120 ºC for 3 min using the TLC Plate Heater. The same samples are also derivatized using the same conditions with α-naphthol and orcinol. For these two last reagents, the derivatization temperature was 110 ºC. All derivatization reagents were prepared as previously described [1]. All data is extracted as previously reported using information from the RGB channels and gray scale [2].
Documentation
Images of the plate are captured with the TLC Visualizer in white light.
Results and discussion
As expected, the information produced by HPAEC was able to differentiate agave specimens according to their species and age. Moreover, the data was also good for creating supervised models. The HPAEC models indicated a decrease of simpler sugars such as fructose, glucose and sucrose, while fructans with higher degree of polymerization (DP) are synthetized as the agave age increases. Interestingly, the visual inspection of HPTLC chromatograms, independent of the derivatization reagent, showed the same trend. Representative chromatograms of agavins from A. potatorum derivatized with aniline, α-naphthol and orcinol showed this trend. Also, it was observed that α-naphthol and orcinol produced more intense monochromatic bands, while aniline produced bicolor patterns. That is, blue zones indicate glucose containing aFOS and pink zones fructose containing aFOS. Using the standards’ RF values, DP-11 was determined as the maximum visible countable DP for aFOS.
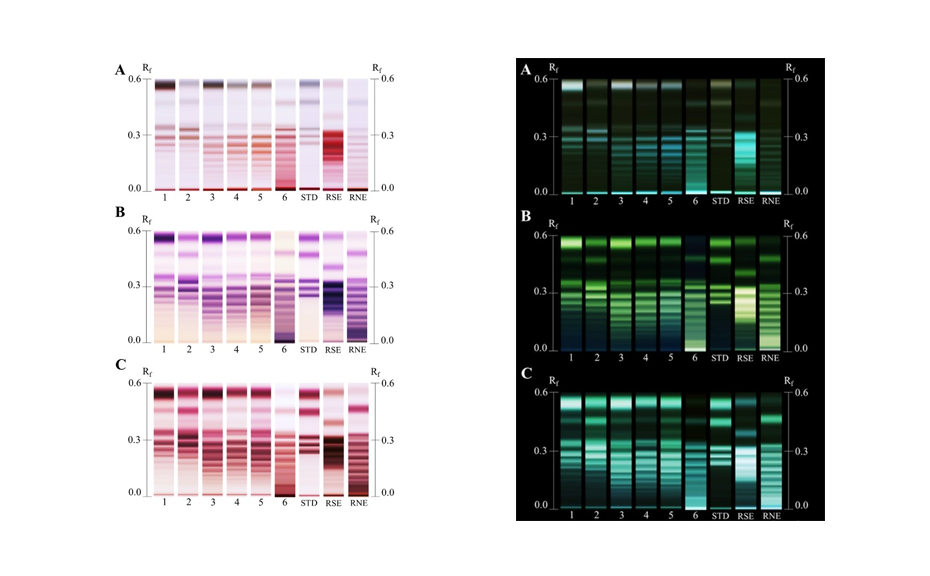
(Left) Processed HPTLC chromatograms in white light (according to [1]) of representative Agave potatorum samples and (right) negative-HPTLC chromatograms, after derivatization with A aniline, B α-naphthol and C orcinol. STD, standard mixture; RSE, raftilose; RNE, raftiline. Track number indicates agave age expressed in years. Reproduced from [1]. (https://creativecommons.org/licenses/by/4.0/legalcode).
For MVDA, the intensity values of the peak profiles from images (PPID) were inverted during data extraction [1] and negative-HPTLC chromatograms were processed with an open-source-software in all color channels according to [2]. The data was normalized to the quality control sample track in each corresponding plate. Furthermore, the data was scrutinized by principal component analysis (PCA), orthogonal projection to latent structures discriminant analysis (OPLS-DA) and orthogonal projection to latent structures (OPLS) analysis. Subsequently, data was approached by MVDA and a PCA showed a clear separation dictated by species factors along the PC1 (captures the most variation), while samples were separated by age along the PC2 (the second most variation). Moreover, there was a clear subgrouping of samples from 1-3 years old plants and 4-6 years old plants. Thus, samples were classified as younger than four years (YT4) and older than three years (OT3), and they were then analyzed by OPLS-DA. The model was well validated through permutation test (100 permutations, Q2 = 0.82) and in a CV-ANOVA test (p = 2.39 x 10-8). The S-plot of the analysis indicated that A. potatorum samples possessed a higher content of glucose/fructose (RF 0.57) and DP5 (RF 0.25 – 0.26) compared to A. angustifolia, which possessed higher contents of sucrose (RF 0.47) and high-DP aFOS (RF 0.04 – 0.13).
To further explore metabolic differentiation correlated to age, the data set was approached by OPLS using agave age as a quantitative “Y” variable. The analysis was well validated (Q2 = 0.98, p = 2.93 × 10-7) and indicated that carbohydrate variation, specially increase of DP-7, DP8 and D-P9 aFOS (RF 0.14, 0.13, 0.11, 0.16, 0.17, 0.18, and 0.10) was correlated with the increase of age in both agave species determined by the Predictive Variable Importance for the Projection (VIPpred)-plot. It is worth to mention, that models resulting from HPTLC data provided higher Q2 and p-values than those obtained from HPAEC data. For instance, the HPAEC model for YT4/OT3 differentiation produced a Q2 = 0.66 and p = 8.13 × 10-15. Furthermore, the same data set produced a Q2 = 0.80 and p = 8.13 × 10-5 for the OPLS model of carbohydrate and age correlation. Here, we only present the best models for each scrutinized factor. Thus, the best PCA model to separate samples according to species and age is that produced from plates derivatized with aniline and extracted in gray channel. The best OPLS-DA model to classify samples according to species is that produced from plates also derivatized with aniline but extracted in blue channel. The best OPLS model for age correlation is produced from plates derivatized with α-naphthol and extracted in green channel.
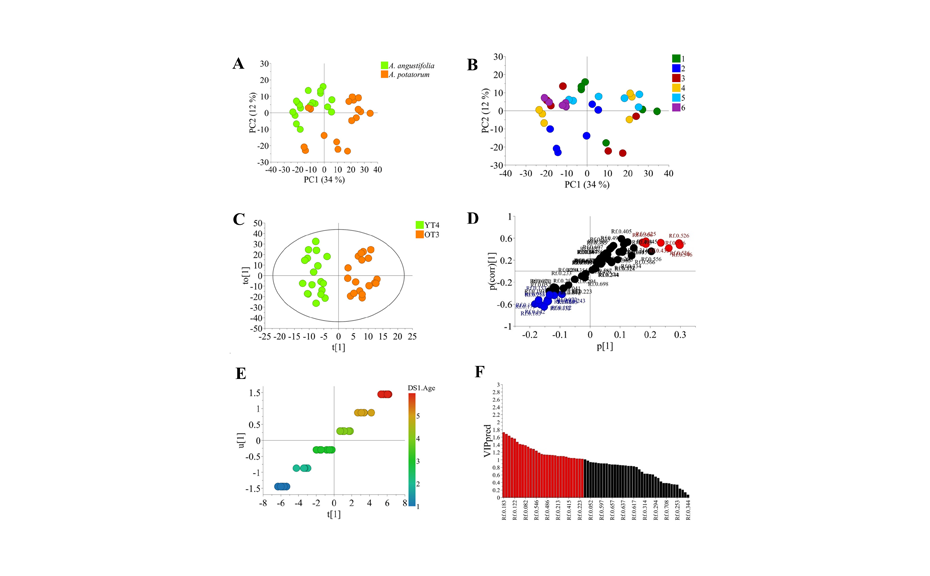
MVDA of HPTLC data approached by A, PCA colored according to species; B, PCA colored according to age; C, OPLS-DA for plants younger than 4 years old and older than 3 years old; D, S-plot of OPLS-DA for YT4/OT3 differentiation; E, orthogonal projection to latent structures for carbohydrate/age correlation; F, Predictive Variable Importance for the Projection (VIPpred)-plot of the age OPLS model. Reproduced from [1].
Thus, we concluded that the aFOS fraction was enough to describe agavins metabolism and that HPTLC data was robust enough to be combined with MVDA, which produced even better supervised models than HPAEC.
[1] L.F. Salomé-Abarca et al. (2023). Curr. Res. Food Sci. 100451, https://doi.org/10.1016/j.crfs.2023.100451.
[2] D. Fichou et al. (2016). Anal. Chem. 12494, https://doi.org/10.1021/acs.analchem.6b04017.
Further information is available on request from the authors.
Contact: Dr. Mercedes G. López, Department of Biotechnology and Biochemistry, CINVESTAV-Irapuato, 36824, Guanajuato, Mexico, mercedes.lopez@cinvestav.mx