The Jean-Pierre Bourgin Institute (IJPB) is the largest center for plant biology at the National Research Institute for Agriculture, Food and Environment (INRAE). It combines resources and multidisciplinary skills in the areas of biology, chemistry, and mathematics. Located in Versailles, it is a Joint Research Unit between INRAE and AgroParisTech. The DYSCOL (Dynamics and structure of Lipid Droplets) team studies various aspects of lipid accumulation in seeds from oil crops and model plants. The Kennedy pathway allows storage of fatty acids in eukaryotes in the form of triacylglycerols (TAG), through successive acylation of a glycerol backbone by acyltransferases. Hundreds of different fatty acids are found in plants. The different fatty acids incorporated into vegetable oils confer them specific physical, chemical and nutritional properties. DGATs (diacylglycerol acyltransferases) incorporate the final fatty acid in position sn-3 of the glycerol skeleton. They catalyze the rate-limiting step of the whole pathway. Due to their impact on oil yield and quality, DGATs are targets of interest for oil engineering. We aimed to identify candidate proteins as DGATs and to understand their substrate specificity. We used various approaches based on recombinant protein expression in different hosts, and analysis of the products of the reaction by complementary approaches, GC and HPTLC.
Introduction
Historically, demonstration of DGAT activity used radioactive precursors of substrates [1] followed by tedious extraction of the products (TAGs) [2] and quantification by liquid scintillation counting. Trans-methylation of TAGs produces fatty acid methyl esters, subsequently extracted, then separated and analyzed by GC. Alternatively, extraction of TAGs by improved methods and their separation from other cellular constituents by TLC is a convenient method avoiding radioactivity. Derivatization permits direct identification and quantification of the TAGs by comparison with standards.
HPTLC represents a valuable improvement of TLC. 12–15 samples are routinely separated at the same time on one plate. The method exhibits high sensitivity and reproducibility, and uses low amounts of organic solvents (< 50 mL for one run). Herein, we describe two methods to evidence DGAT activity and specificity using HPTLC.
Method (1) allows the analysis of extracted lipids by derivatization with phosphomolybdic acid reagent. Method (2) is used to study the activity and specificity of a purified DGAT.
Standard solutions
- Solutions of cholesteryl oleate, oleic acid methyl ester, trioleine, oleic acid, cholesterol at 2.7 μg/μL each in CHCl3 are prepared.
- NBD-DOG (1-{N-[(7-nitro-2-1,3-benzoxadiazol- 4-yl)-methyl] amino-decanoyl-2-decanoyl-sn-glycerol) stock solution in chloroform – methanol 2:1 (V/V) at 67.5 ng/μL (between 5.5 ng to 2.7 μg are applied to generate a calibration curve).
Sample preparation
- Lipids are extracted from yeast biomass according to Folch [2], dried under nitrogen, and resuspended in 200 μL of chloroform – methanol 2:1 (V/V).
- DGAT assay to investigate enzyme specificity: NBD-DOG as a fluorescent DAG acceptor and different acyl donors (lauroyl-CoA, palmitoyl-CoA, stearoyl-CoA, oleoyl-CoA, and linoleoyl-CoA. The reaction is carried out at 31°C for one hour under shaking, then stopped by the addition of chloroform – methanol 2:1 (V/V).
Chromatogram layer
HPTLC plates silica gel 60 (Merck), pre-washed with isopropanol, are used.
Sample application
Between 3.0–6.0 μL for standard solutions and 50.0 μL for sample solutions (corresponding to 400 μg cells, dry weight) are applied as bands with the Automatic TLC Sampler (ATS 3), 15 tracks, band length 5.0 mm, distance from left edge 15.0 mm, distance from lower edge 8.0 mm.
Chromatography
Plates are developed in the Automatic Developing Chamber (ADC 2) with chamber saturation (with filter paper) for 20 min, (1) development with diethyl ether – hexane –methanol – acetic acid 60:40:5:1 (V/V) to the migration distance of 80 mm (from the lower edge), drying for 20 min, (2) development with hexane – diethyl ether – acetic acid 80:20:2 (V/V) to the migration distance of 80 mm (from the lower edge), drying for 20 min.
Post-chromatographic derivatization
The plates are immersed in 5% phosphomolybdic acid in ethanol, then incubated for 30 min at 100 °C using an oven.
Documentation
Images of the plates are captured in white light.
Densitometry
Fluorescence measurement is performed with the TLC Scanner 3 (excitation at 473 nm and emission > 510nm). DGAT activity is expressed as picomoles of TAG formed per minute and per milligram of purified protein, using a calibration curve based on the fluorescent signal of NBD-DOG.
Results and discussion
The following figure shows the results of the separation of lipids extracted from three yeast strains (method 1). The control yeast strain was transformed with an empty vector; a second strain was transformed with the AtDGAT1 sequence encoding for Arabidopsis thaliana DGAT1, and the last strain was transformed with the EgDGAT1 sequence encoding for Elaeis guineensis DGAT1-1, a putative DGAT [3]. Both cassettes encoding for plant DGAT1 restored TAG accumulation in the Yarrowia lipolytica mutant strain [4]. Thus, we conclude that EgDGAT1 sequence was encoding for an active E. guineensis DGAT1-1.
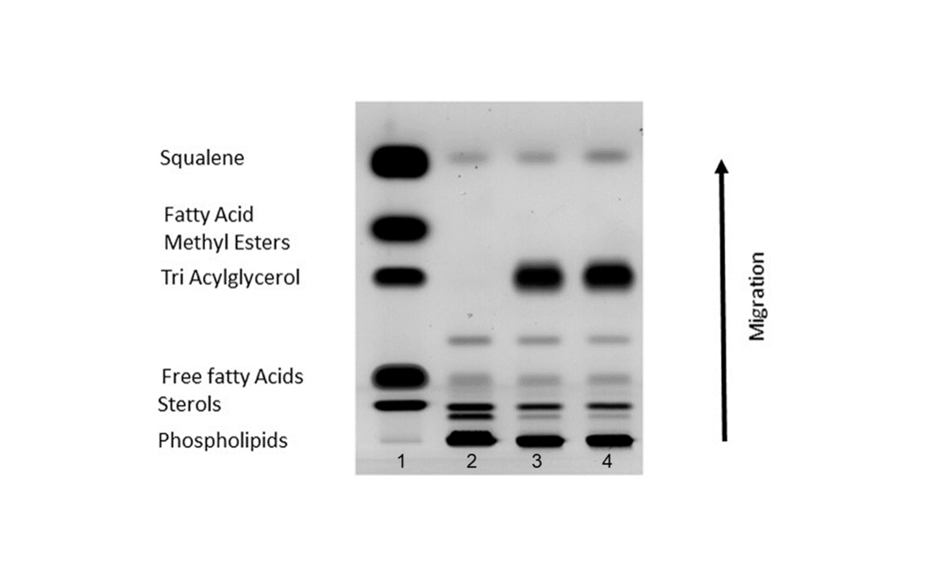
Figure 1: Separation of lipids extracted from yeasts strains expressing plant type 1 DGATs (1: standards, 2: empty cassette, 3: AtDGAT1, 4: EgDGAT1-1).
To generate a calibration curve (method 2), the fluorescence of the NBD-DOG standard applied in different amounts is measured. The standard curve is depicting the dependence of the intensity of the fluorescence of NBD-DOG (minus blank value).
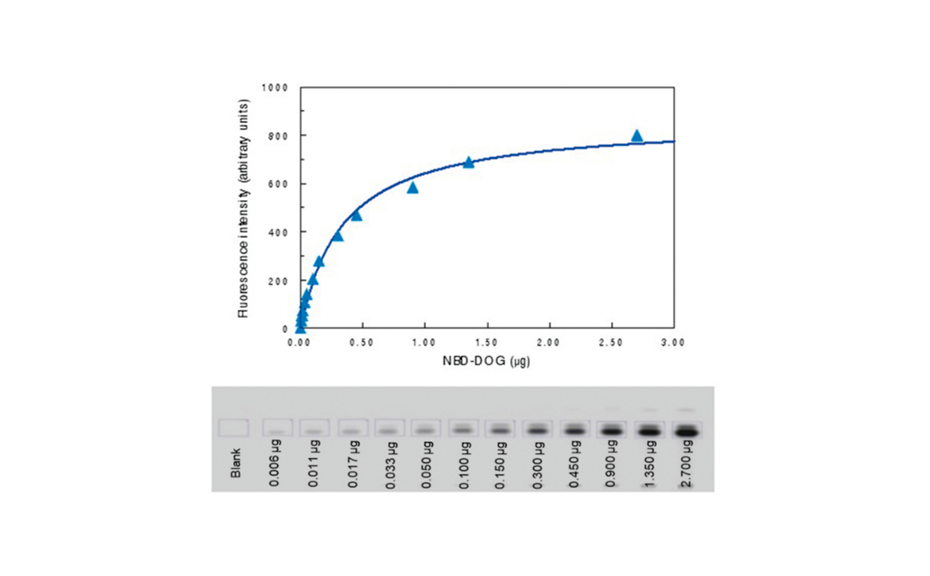
Figure 2: Calibration curve of NBD-DOG (scanned at 473 nm in fluorescence mode) depicting the dependence of the intensity of the fluorescence of NBD-DOG (top) as function of the amount separated on HPTLC plate (bottom)
In another experiment, purified recombinant DGA1, a type 2 DGAT from the yeast Yarrowia lipolytica was incubated with NBD-DOG and acyl-CoA with various acyl chain lengths (C12:0, C16:0; C18:0).
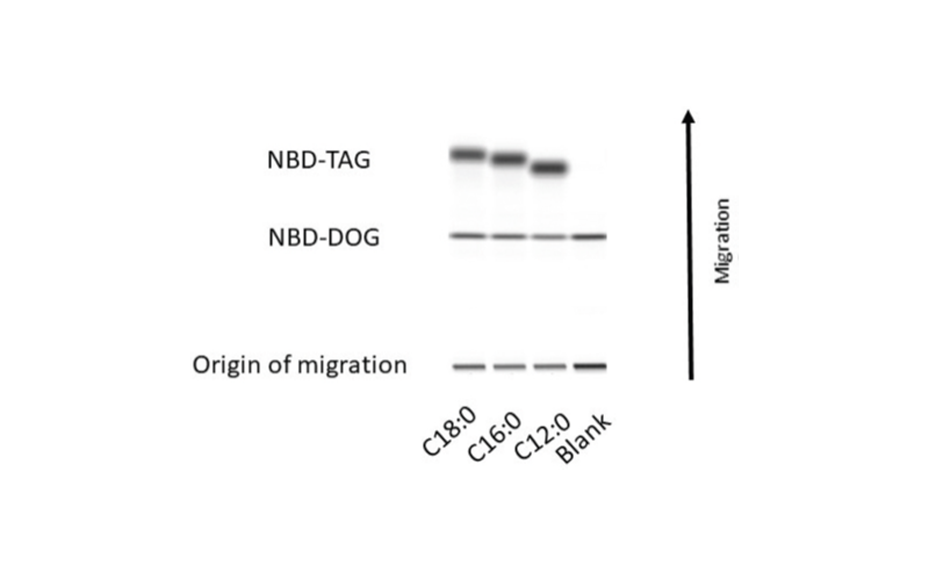
Figure 3: Separation of TAG synthetized by purified recombinant yeast type 2 DGAT (DGA1) using acyl-CoA with different chain lengths.
In the absence of an acyl donor, no NBD-TAG was formed (blank track). In the presence of acyl donors (C12:0, C16:0; C18:0–CoA), NBD-TAGs were formed. Noticeably, the migration of the reaction product depended on the length of the acyl chain incorporated. NBD-TAG with C18:0 migrate over a longer distance by comparison to NBD-TAG with C16:0 or C12:0.
HPTLC is a fast (within two hours), reproducible, and robust method to evidence acyltransferases activities. In vivo (1), complementation of microbial strains affected in neutral lipid metabolism by sequences coding for DGAT led to accumulation of TAGs. The products were extracted, separated by HPTLC and identified directly by comparison with standard molecules. In vitro (2), DGATs transferred various acyls to fluorescent DAG acceptors. Fluorescent products of the reaction (TAGs) were quantified using a substrate calibration curve. The method was sensitive enough to distinguish TAGs differing by only two carbons [5]. Both approaches avoided the use of radioactive labeled products.
[1] Erickson, S.K. and Fielfing, P.E. J Lipid Res 27 (1986) 875–883
[2] Folch, J. et al. J Biol Chem 226 (1957) 497–509
[3] Aymé, L., et al. PLoS One (2015) 10: e0143113
[4] Beopoulos, A., et al. (2012) Appl Microbiol Biotechnol 93: 1523–1537
[5] Haili N., et al. (2016) J Biol Chem 277: 6478–6482
Further information is available on request from the authors.
Contact: Dr. Laure Aymé and Dr. Thierry Chardot, Institut Jean-Pierre Bourgin, INRAE, AgroParisTech, Université Paris- Saclay, 78000, Versailles, France, laure.ayme@recherche.gouv.fr, thierry.chardot@inrae.fr