It is widely accepted that High-Performance Thin-Layer Chromatography (HPTLC) is the method of choice for the analysis of substances in complex matrices involving herbal drugs and herbal drug preparations. High-end instrumentation and standardized procedures enable HPTLC to deliver reproducible and cGMP-compliant results.
The publication of general chapters on HPTLC for the identification of plants and extracts as part of monographs of the United States Pharmacopoeia (USP-NF 2015) and the European Pharmacopoeia (Ph. Eur. 2017) emphasizes today’s role of HPTLC for the identification of samples from botanical origin.
HPTLC delivers a chromatographic fingerprint of the sample in its entirety, a feature that makes the method ideally suited for the analysis of botanical materials, herbal drugs and herbal drug preparations, all of which are highly complex and consist of many different components. Apart from this, the exact chemical composition of botanical products is unknown and may vary widely, both qualitatively and quantitatively.
The quantitative content of known active or inactive components in herbal drugs is not sufficient as a quality criterion: the presence of other substances must also be part of the analysis of a sample.
Why use HPTLC?
The advantages offered by HPTLC add up to a compelling argument for choosing it as an analytical technique.
Visual output
HPTLC is the method of choice for the analysis of substances in complex matrices. The HPTLC fingerprint of herbal drug samples visually either confirms or rejects the plant identity. HPTLC is a highly flexible analytical technique and allows adapting the analytical method to the individual needs in each process step. For each sample, the separated analytes remain on the plate and allow for further post-chromatographic processing.
Multiple detection of separated analytes
Post-chromatographic derivatization permits the use of additional detection modes and makes differences in fingerprints clearly visible. In contrast to other chromatographic techniques, the separated analytes of the sample remain on the plate.
Analysis of multiple samples in parallel without cross-contamination
HPTLC allows for parallel instead of sequential analysis with little to no sample preparation. At least 15 samples can be developed and then analyzed in parallel under identical conditions at the same time. Due to single use of the plate, there is no risk of cross-contamination.
Cost-efficiency
HPTLC offers short run times per sample (e.g. a total analysis time of 30 min for 15 samples means a run time of 2 min/sample) and low solvent consumption per sample, making it a highly cost-effective form of analysis.
Flexibility
HPTLC is an open system, enabling to set and optimize all influencing parameters independently of each other, and offers a nearly unlimited choice of mobile phases.
Disposable plates
HPTLC plates are disposed of after use, eliminating the problems caused by samples with high matrix content, which may block HPLC columns and cause ghost peaks.
Coupling to Mass Spectrometry
MS hyphenated with HPTLC is a powerful additional detection tool and allows the confirmation of identity of targeted analytes. High-resolution MS also enables some structure elucidation.
Compliance
HPTLC instrumentation from CAMAG can be used in a cGMP/cGLP environment. Additionally, software-controlled HPTLC instruments support full compliance with 21 CFR Part 11.
Identification of raw materials and products
HPTLC generates a chromatographic fingerprint of the drug sample in the form of a unique sequence of zones or peaks or due to the components of the sample (as illustrated by the chromatogram of green tea). The fingerprint of botanically authenticated raw material serves as a primary reference against which unknown materials can be characterized. Both, sample and reference material are chromatographed side by side on the same plate. The resulting fingerprints are then compared with respect to the number, sequence, position and color of separated zones.
Example: HPTLC fingerprints of green tea extract and other caffeine containing botanicals
The polyphenol fingerprint of green tea differs significantly from that of other caffeine containing botanicals (top). In UV 254 nm prior to derivatization (bottom), caffeine can be detected.
Conclusion
HPTLC is a reliable technique for identification of green tea extracts based on polyphenols. During the same analysis, the caffeine content of the material can be determined.
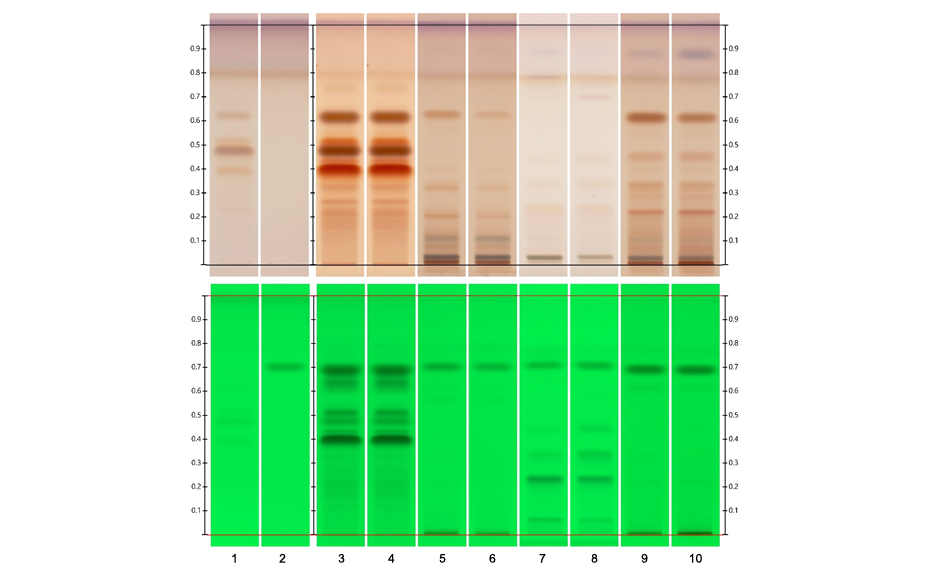
Figure 1 : Track assignment – 1 reference substances with increasing RF: epigallocatechin gallate, epigallocatechin, epicatechin gallate, and epicatechin; 2 caffeine; 3–4 green tea extracts, 5 Cola nitida seed (red), 6 Cola nitida seed (white), 7 Coffee been (roasted), 8 Coffee been (green), 9–10 Guarana seed
Detecting Adulteration
One problem commonly encountered when controlling the quality of botanicals is the intentional substitution or inadvertent confusion of plant species. Adulteration or falsification becomes critical if the undesired plant species are toxic. Any method used for identification purposes must thus be specific and sufficiently sensitive.
Example: Controlling the quality of Stephania tetrandra
Stephania tetrandra is often confused with or replaced by toxic species of the Aristolochia genus, such as Aristolochia fangji. The very similar Chinese names of the two species are an additional source of confusion. HPTLC can detect the presence of aristolochic acids (AA) down to a concentration of 1 ppm, which means that the adulteration of Stephania with as little as 1% Aristolochia is visible.
Conclusion
HPTLC is a sensitive, rapid and cost efficient technique for detecting adulteration of Stephania tetrandra with aristolochic acids.
An HPTLC-based limit test for aristolochic acid is proposed by the European Pharmacopoeia (chapter 2.8.21).
Quantification of marker compounds
The most commonly used measure of sample quality is the amount of specific active compounds and/or marker compounds. Those are best quantified using either scanning densitometry or image-based evaluation.
Example: Densitometric quantification of oleuropein in Olive leaf dry extract
Oleuropein is a polyphenolic compound often used as marker for extracts and products derived from olive leaf.
Conclusion
HPTLC with scanning densitometry facilitates spectrally selective, sensitive, and precise quantification of substances in plant material. With comprehensive HPTLC fingerprinting, quantitative information about substances is available via peak profiles from images (PPI).
Other applications
Product development
HPTLC is used to optimize process parameters and detect changes and degradations in the material during formulation. It is a particularly effective type of analysis because it is fast and can be applied to many different samples simultaneously.
Process control
HPTLC is ideal for demonstrating the consistency of product quality because it proves that raw materials retain their integrity and that no decomposition takes place during production.
Stability tests
Thanks to the instrumentation and standardization offered by HPTLC, it is possible for the same process to be repeated over a prolonged period of time. This makes it a suitable method for stability tests in which the samples are compared from plate to plate over protracted periods. The first plate serves as the reference with which all subsequent plates are compared. Another significant advantage is that a large number of different samples can be analyzed quickly, making HPTLC a fast, efficient solution for stability tests.